Bridging the Gap: From Massive Stars to Supernovae in 3D
Oxygen shell burning
Figure 1: Overturn motions in the oxygen burning shell of an 18 solar mass star involving plumes of unburnt oxygen (green) and silicon ashes (red) around the silicon-iron core of the star (cyan). Part of the star is removed for better visibility.
Massive stars die catastrophic deaths. Once they have exhausted their nuclear fuel at the centre, the innermost part of the star, an iron core about 1.5 times as massive as the sun, succumbs to gravity and collapses to an ultra-dense neutron star within a fraction of a second. In the process, the outer layers of the star are expelled in a gigantic supernova explosion with velocities of thousands of kilometres per second.
Such supernovae are regularly observed in distant galaxies, and within the Milky Way we can still see the debris sits of many such explosions hundreds and thousands of years later. Much of the world around us is, in fact, ultimately debris from massive stars - from the oxygen we breathe to the calcium in our bones. But a puzzle remains: How is the collapse of the star turned into an explosion?
Stellar collapse with explosion
Figure 2a: Slices through the core of a massive star after collapse. The neutron star is visible as the dark-blue region in the centre, the neutrino-heated matter behind the supernova shock is shown in red. Due to the infall of the asymmetric oxygen-silicon layer, the shock starts to expand at around 0.3 seconds.
The most promising theory posits that extremely light and weakly interacting elementary particles, called neutrinos, are key in this process. These are emitted copiously from the surface of the young neutron star, which is a few thousand times hotter than the centre of the Sun. Part of these neutrinos are absorbed by matter falling onto the neutron star, heating it up. If the heating is sufficiently strong, the collapse is reversed, and the neutrino-heated matter drives an expanding shock wave through the star.
Theorists have long attempted to show that this idea works with the help of computer simulations. But even though one can now simulate the collapse of massive stars in three dimensions (3D), the computer models often still fail to explode. The international team of researchers including MPA scientists has now worked on a solution to this problem by invoking more realistic initial conditions. The team replaced the spherical stellar models, from which supernova calculations were previously started, by fully three-dimensional initial data.
Stellar collapse without explosion
Taking into account the asymmetries that exist in the progenitor star prior to its collapse enabled a neutrino-driven supernova explosion. The astrophysicists could follow the evolution of the expanding blast wave in a continuous, consistent 3D calculation for the longest period to date. This breakthrough in our understanding of the highly complex processes that lead to the explosion of massive stars has become possible by using supercomputers in Australia, Germany, and the UK.
For a successful explosion in 3D it is crucial that there are already violent overturn motions before collapse driven by nuclear fusion, which need to be stirred even more to trigger an explosion. To explore this possibility, the team simulated the fusion of oxygen to silicon in an 18 solar mass star for the last six minutes before the star reached the end of its stable evolution (see Figure 1). The researchers found that they could obtain a successful explosion only because the collapsing silicon-oxygen shell was perturbed by vigorous mass flows already. They then followed the beginning supernova for more than two seconds (see Figure 2).
Supernova shock wave
Figure 3: Expansion of the neutrino-heated matter (yellow/red) and the supernova shock wave (translucent cyan surface) during the explosion of an 18 solar mass star.
It still takes about a day until the shock front reaches the surface of the star and the stellar debris is expelled into the surrounding space; nevertheless the computer model already is able to tell that the explosion and the relic neutron star are starting to look like the ones observed: The explosion produces about 0.06 solar masses of iron-group elements. The neutron star is about 1.7 times as massive as the Sun. It rotates once every 20 milliseconds, and it is kicked away at a speed of 600 kilometres per second because the explosion is strongly asymmetric. This means that the simulations cough up a plausible explosion model – without tweaking.
Now that such successful stellar explosion simulations are feasible, the team will systematically explore how supernovae from different progenitor stars look like. More 3D calculations are needed to clarify which stars blow up by the crucial aid of pre-collapse perturbations in the convective burning shells around the stellar iron core.
Bernhard Müller, H.-Thomas Janka, Tobias Melson, Alexander Heger
Acknowledgments:
This project was partly funded by the European Research Council through grant ERC-AdG No. 341157-COCO2CASA and the Australian Research Council (FT120100363, FT120100363). Computing time was kindly provided by the Gauss Centre for Supercomputing on SuperMUC (GCS@LRZ, Germany) and by NCI Australia and the Pawsey Supercomputing Centre.


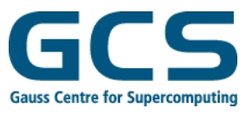