Probing the nature of the most luminous explosions
Supernovae are extremely bright stellar explosions – superluminous supernovae are even brighter. However, the nature of these most luminous explosions has remained a mystery. In a new study, MPA researchers now present their simulations of superluminous supernova spectra months and even years after the outbreak and show that they are very similar to gamma-ray bursts, another type of highly energetic explosions. In addition, the results point to very high masses of oxygen and magnesium, suggesting very massive progenitor stars that will use an exotic explosion mechanism rather than the standard neutrino-driven explosion believed to power most supernovae.
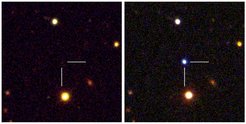
These two images show observations of a superluminous supernova detected by the Palomar Transient Factory project in 2009 (PTF09cnd, z= 0.258). The pre-explosion image is from Sloan Digital Sky archive data, the post-explosion images are composed from observations made with the Palomar Observatory’s 1.5-m telescope, the Wise Observatory’s 1.0-m telescope and the Ultraviolet/Optical Telescope on board NASA’s Swift satellite.
Superluminous supernovae are a new and exotic class of stellar explosions, radiating up to 100 times more energy than normal supernovae. Despite being so bright, they were discovered only about 10 years ago, as they occur at large distances and are quite rare (one per every thousand normal supernovae).
The origin of the enormous luminosity and the properties of the progenitor stars have been shrouded in mystery. They may be powered by rapidly spinning and highly magnetized neutron stars (so called magnetars), accretion onto a newly formed black hole, huge amounts of radioactivity, or violent collisions with dense circumstellar matter. What type of progenitor stars give rise to them? Why do they occur exclusively in unusual dwarf galaxies?
In a new study led by Dr Anders Jerkstrand, a Marie Curie Fellow at MPA, several important new advances are presented, which are based on calculating spectral models of supernovae. “Several months and years after the supernova has exploded, when the ejected material expands and cools, the spectra reveal signatures of the elements that have been produced inside the star,” Jerkstrand explains. “By comparing observed to modelled spectra in this phase, we can get an insight into the inner layers of the progenitor, which in turn provides strong constraints on the origin and nature of these explosions.”
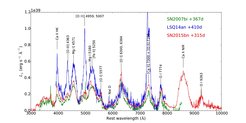
Interpretation of the spectra requires sophisticated models of how radiation passes through the expanding gas and requires the latest atomic physics to be included in the detailed models. What made this study unique was the combination of state-of-the-art new models applied to the highest-quality data ever collected on these supernovae at such late times by the PESSTO survey with the European Southern Observatory's facilities.
The study reveals the first clear picture of the chemical composition of these explosions. The new spectra are demonstrated to have strong similarities with gamma-ray burst supernovae, the first time this link has been established. Gamma-ray burst supernovae are thought to arise by the formation of a black hole that punches a relativistic jet through the infalling star, or by the formation of a highly magnetic neutron star. Gamma ray bursts are similarly rare as superluminous supernovae, and also occur in irregular dwarf galaxies at low metallicity. Some of them are actually accompanied with supernovae, but until now always at much lower luminosities, and not lasting as long as superluminous supernovae.
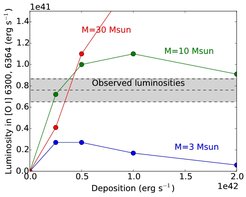
This figure shows the observed oxygen line luminosities (gray band) compared to models with different oxygen-zone masses (3,10 and 30 solar masses). This illustrates that the oxygen mass has to be fairly high to match the observations over a broad range of energy inputs.
In a second important discovery, the spectral synthesis models revealed that these superluminous supernovae contain among the highest oxygen masses inferred for any supernova so far. The spectra show very strong emission lines requiring more than about 10 solar masses of oxygen and 1 solar mass of magnesium. These explosions must therefore come from extremely massive stars, with over 40 solar masses on the main sequence. Stars in this mass range are unlikely to explode with the large inferred kinetic energies by the standard neutrino-driven mechanism, and a more exotic mechanism such as a magneto-rotational driven jets or black hole accretion is needed.
Detailed multi-dimensional models involving the collapse, explosion, and late-time energy input of the massive stellar core are currently being pursued by several groups around the world. Together with the new constraints derived in this study, this promises to expand our knowledge of stellar evolution and supernova explosions into new and unexplored regimes.

This work has been supported by the European Union’s Framework Programme for Research and Innovation Horizon 2020 under Marie Sklodowska Curie grant agreement No 702538, and by the Seventh Framework programme ERC grant No 291222.