Constraining theories of gravity using the large-scale distribution of galaxies
Dark energy and modified gravity
Almost twenty years ago, astronomers observed that the light emitted by distant Type Ia supernovae explosions is even fainter than was expected. This extra dimming provided the first evidence for the accelerated expansion of the Universe. Soon after these observational data were presented to the astronomical community, it became clear that an explanation would require new physics. One way out of the problem consists in adding a new term to Einstein’s field equations: some exotic forms of ”dark energy” or a cosmological constant, which act as sources that drive the accelerated expansion. Another explanation consists in noting that General Relativity is not nearly as well tested on cosmological scales as it is in our Solar System, and that the acceleration could be simply due to the different nature of gravity on very large scales. This has motivated several recent studies on modified gravity models (as non-GR theories are collectively known) and their cosmological predictions; making this one of the most active areas in cosmology today.
Braneworld gravity models
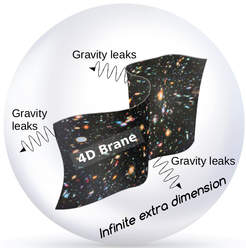
Figure 1: Schematic representation of the DGP brane model. Matter and radiation are confined to the four-dimensional brane, but the gravitational interaction is free to propagate out into the additional fifth dimension.
One of the most popular modified gravity scenarios is that of "braneworlds". In these theories, the four-dimensional spacetime we live in (3 space + 1 time dimension) is just a slice, or "brane", of a higher dimensional spacetime. One concrete example is the Dvali-Gabadadze-Porrati (DGP) model, where we live in a four-dimensional brane of a five-dimensional "bulk" spacetime.
Figure 1 shows a cartoon that summarizes the idea behind these models: matter and radiation are confined to the brane, but the gravitational interaction can "leak" out of the brane into the higher dimension(s). This leakage modifies how gravity operates in the brane, thereby permitting deviations from General Relativity to occur. A crucial point to bear in mind is that the modifications to General Relativity should only occur on distance scales much larger than the Solar System, otherwise these models would be immediately ruled out by the very precise tests performed in our solar neighbourhood, which confirm the validity of General Relativity extremely well.
Measuring the growth rate of structure
Cosmologists have defined the growth rate of structure as a quantity that measures how fast large lumps of matter merge with one another in the Universe causing structure to gradually grow over cosmic time. The stronger the gravitational interaction, the faster the growth rate will be. Precise and accurate determinations of the growth rate therefore are a very good probe to test gravity.
The growth rate however cannot be directly measured by pointing a telescope at the sky. Instead, it can only be determined through complex and advanced modelling of the observed clustering pattern of galaxies on large scales. A worry then emerges: if the processing of the galaxy clustering data is faulty, the resulting growth rate measurements are shifted away from the true value. Consequently, we would be drawing wrong conclusions about the various theories of gravity. Therefore, to guard against this worry, the modelling techniques for real data are validated against mock observations based on N-body simulations of structure formation in the Universe. In this way, we can test the validity of the observational pipelines in a controlled setup, in which we know the real answer.
A major shortcoming of previous validation tests was that they were only applied to simulations based on General Relativity. The validity of the same techniques with respect to other theories of gravity remained uncertain. Researchers at MPA and MPE have now, for the first time, undertaken a thorough validation of these observational analysis pipelines by testing them on mock observations constructed from N-body simulations of DGP brane models with varying degrees of departure from General Relativity. The mock galaxy catalogues were designed to be fair descriptions of the galaxy samples from the BOSS survey, the largest galaxy survey to date in terms of the number of galaxies and the volume of the Universe covered.
Tight constraints on DGP gravity
First, the scientists ensured that the modelling steps for determining the growth rate from the data remain valid even in the case of DGP gravity. Then, the authors used the latest data from the BOSS survey to constrain the model. In doing so, it has become standard to focus on a subset of the DGP gravity model to place benchmark constraints on departures from General Relativity. This standard toy model is known as nDGP ("n" for normal) and automatically passes Solar System tests of gravity as well as constraints from geometrical probes sensitive to the expansion rate of the Universe.
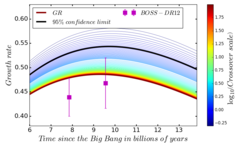
Figure 2: Growth rate as a function of cosmic time. The purple squares show the measurements from the final Data Release 12 of the BOSS galaxy survey. The thin lines are colour coded by the crossover scale, please note that the scale is logarithmic. The thick black line shows the result for crossover scale ≈ 1, which marks the so-called 95% confidence limit: given the data, the "true" curve is below the thick black one with 95% probability. This represents the tightest observational constraint on this model to date. The thick red line shows the result from General Relativity.
The main source of constraining power therefore is the data which probes structure formation on large scales. Figure 2 illustrates how the growth rate data can constrain the nDGP model. The growth rate is shown as a function of cosmic time for several values of the so-called "crossover scale", a parameter that basically determines the distance above which gravity starts to leak out of the brane. Smaller values of the crossover scale lead to stronger gravity, pushing the prediction away from the data, while larger values bring the nDGP model closer to General Relativity – and to a better agreement with the measurements from BOSS.
One of the main conclusions of this work is that, reassuringly, current observational methods do not show evidence for a biased performance when tested on mock universes with nDGP gravity. This constitutes a very much needed test of the validity of current observational analyses, which ensures that current data on the growth rate can be used to test the nDGP model (as well as a plethora of other theories with similar phenomenology).