Unveiling the Universe at the field level
The distribution of galaxies on large, cosmological scales holds important clues on the nature of dark matter, the properties of dark energy and the origin of our Universe. Yet, optimally retrieving this information from observations is challenging. MPA researchers are developing a novel analysis approach, where they follow the evolution of cosmic structures through their entire formation history. Enabling a very detailed comparison between theoretical models and observational data, this approach will allow measuring key parameters of dark matter and dark energy very precisely.
The spatial pattern of galaxies in the Universe exhibits a complex, web-like structure with large galaxy clusters at the nodes that is interspersed by fast voids. Cosmologists have developed a detailed understanding how this cosmic large-scale structure emerged. In a nutshell, an early inflationary period of rapid expansion created very small (of order 1 in 100,000) fluctuations in the cosmic energy density, which subsequently evolved under their self-gravity as the Universe expanded. Yet, in this picture, there remain several open questions:
- What is the nature of dark energy that drives the accelerated expansion of the Universe?
- What is the nature of dark matter, whose presence is required by gravitational dynamics?
- What is the physical mechanism that drives inflation?
Dark matter is about five times more abundant than ordinary, “visible” matter. General Relativity requires visible and dark matter to experience the same gravitational forces, so the evolution of dark matter fluctuations can be predicted very precisely. It depends sensitively on the interplay between gravitational attraction and the cosmological expansion. Therefore, the cosmic large-scale structure holds important clues on the fundamental questions about our Universe. Large cosmological surveys map the distribution of galaxies out to very large distances. Yet, these galaxies comprise only the “tip of the iceberg” while the majority of the cosmic large-scale structure resides in dark matter and remains invisible.
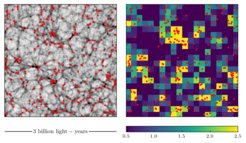
Is there a way to deduce the distribution of dark matter in our Universe from the observed positions of galaxies? Can we understand how the structures we see today have formed? And can this help us to better answer the fundamental questions we have about our cosmos? The answer is yes. However, as we will see, we first need to overcome some challenges.
First, galaxies do not follow the distribution of matter one-to-one, we only observe a limited number of them, and measurement uncertainties affect the observations. Thus, the matter distribution that explains the observations is not unique – there can be multiple possibilities. A statistical Bayesian analysis can capture this uncertainty: we generate several plausible matter distributions, evaluate how consistent they are with the observations, and only keep those that show the highest level of agreement.
The relation between galaxies and the underlying matter density field is modelled with a theoretical framework called the Effective Field Theory (EFT) of Large Scale Structure. This effective theory has a number of free parameters designed to make our results insensitive to the details of galaxy formation on small scales, which we cosmologists do not yet understand completely. Moreover, the theory gives us a “likelihood” that quantifies the level of agreement between a proposed matter distribution and the observed galaxies. Since this comparison is done voxel by voxel (3D pixels), this is also known as a field-level analysis.
The second challenge is how to propose plausible matter distributions in the first place. The matter distribution in the universe today is the result of a complex dynamical evolution. In contrast, the distribution of primordial perturbations, which provide the starting point to this evolution, have simple statistical properties. We can take a guess on the primordial density, forward model their evolution in time, and compare that prediction to the observation. We repeat this over and over to discover the configurations best explaining the data and explore all possible solutions.
Each possible realization of the three-dimensional primordial density is characterized by up to a million parameters. And for each of these realizations we have the full history of structure formation. To explore this huge range of possibilities efficiently, we have developed our own numerical code LEFTfield, which is heavily parallelized, provides gradient information and runs on computing clusters. It can generate plausible matter distributions and forward evolve them through cosmic time in just a few seconds. Figure 2 illustrates the result of such an analysis on a simulated dataset, where each frame of the animation shows a separate simulated universe that is compatible with the observation. The observations best constrain the distribution of matter on large scales. Correspondingly, the animation shows little variability in the inferred density for larger structures, but more movement in smaller ones.
How does this help to answer the questions posed above? The properties of dark matter and dark energy are built into our numerical forward model for structure formation. We can now modify these parameters and see how the agreement with the data changes. For example, changing the amount of dark energy or how it evolves leads to a different expansion rate of the universe, which in turn affects the growth of structure. This comparison therefore allows us to measure key parameters of dark matter and dark energy.
The field-level analysis extracts all information available in the large-scale galaxy distribution; our ultimate goal is to achieve a much more precise measurement of dark matter and dark energy than current state-of-the-art analyses. The latter only compare theory and data at the level of highly-compressed summary statistics (such as the RMS fluctuations as a function of scale). A further advantage of forward modeling is that we can incorporate observational and instrumental effects present in cosmological surveys. One example are redshift-space distortions from the peculiar motion of galaxies. In fact, the peculiar motions allow us to extract additional cosmological information, as they directly probe the growth rate of cosmic structures.
We are continuing to advance the technique to apply it to state-of-the-art cosmological observations, where they will hopefully allow us to better understand dark matter and dark energy. A comparative analysis that we performed recently shows that field-level analysis indeed yields tighter measurements than the standard approach, so stay tuned for some exciting highlights we hope to share soon.