A Universe made of Black Holes?
The nature of dark matter is still very much unknown; viable candidates range from microsopic elementary particles to black holes with masses many times that of the Sun. Researchers at MPA, Carnegie Observatories, and the University of Sussex have recently made concrete and reliable predictions for how the Universe would look if dark matter consists entirely of massive black holes: they performed the first self-consistent study of how structure would form in such a Universe, and how many of these black holes merge and emit observable gravitational waves.
Astronomers and cosmologists have collected a wide range of evidence for the existence of dark matter, a clustering matter component that has so far only been observed via its gravitational effects. Many dark matter candidates have been proposed, from microsopic elementary particles all the way to black holes with masses several times that of the Sun. Black holes comprising the dark matter would have to have formed in the early Universe, and hence are known as primordial black holes (PBH); astrophysically formed black holes (e.g., from stellar collapse) could not explain the evidence for dark matter seen in early-Universe probes, such as the cosmic microwave background, and would not be abundant enough. One possibility is that the PBHs were generated toward the end of the epoch of inflation in the early Universe.
A wide range of observations, including gamma-ray and X-ray backgrounds, gravitational lensing, the large-scale structure of the Universe, and the cosmic microwave background, have placed various constraints on the allowed PBH masses. Nevertheless, at least a fraction of dark matter might still consist of PBHs in several potential “mass windows”.
Researchers at MPA, Carnegie Observatories, and the University of Sussex have recently performed the first self-consistent study of how structure would form in a Universe with primordial-black-hole dark matter (of mass about 16 solar masses), by investigating the evolution of a region in the Universe with slightly above-average density. They carefully treated the evolution throughout the primordial Universe, and then continued the calculation with the state-of-the-art N-body code BIFROST. BIFROST calculates all gravitational forces between each black hole in the simulation without any small-scale resolution limit. It also includes relativistic effects responsible for libration and precession of binary PBH orbits, reminiscent of Einstein’s famous explanation for the periapsis advance of Mercury’s orbit. Most importantly, gravitational-wave radiation reaction effects are also modelled, allowing PBH binaries to merge in the simulations. This pilot study assumed that 100% of dark matter consists of PBHs. Mixed models with various dark matter components including collisional PBHs are numerically far more challenging to simulate, but might become feasible in the next few years.
The right column of Video 1 shows the evolution of the dark matter density in this scenario. For comparison, the team also performed simulations of the same region within a scenario where dark matter is made of microscopic particles (left column). The distribution of particle dark matter is much more smooth, while the discrete massive bodies are visible in the PBH simulation. The middle column shows the result of an approximate PBH simulation, where small-scale gravitational interactions are neglected; the clearly visible differences with the right column illustrate the importance of an accurate code like BIFROST for this study.
The dynamics of the system of black holes is highly complex. Close encounters between multiple black holes can lead to slingshot effects where one black hole is “kicked” with high velocity (the energy for this kick comes from the deep potential wells of the black holes). This is illustrated in Video 2, which shows the motions of individual black holes in the simulation over a short period of time (essentially, a dynamic, zoomed-in version of Video 1). Capturing these dynamics accurately while also being able to follow the system over the Universe’s history (or at least a substantial fraction of it) is very challenging, and this study presents the first such calculation ever performed.
Given the dramatic differences between the primordial-black-hole and particle-dark-matter scenarios in Video 1, one would expect that astronomers could easily tell which scenario corresponds to the real Universe. However, we cannot observe either particle dark matter or black holes directly; moreover, the scales shown here are very small and difficult to probe. Thus, a bit of creativity is required to come up with observational routes to probe primordial black holes. For example, the “grainy” mass distribution in the primordial-black-hole case can affect (“heat”) the distribution of stars in galaxies.
One of the cleanest, and probably the most exciting probe of this scenario however are the gravitational waves emitted by the black hole population, in particular when two black holes merge, which happens when they get sufficiently close to each other. This is the subject of ongoing followup work by the team. A first result was already published in the study reported on here, however: the total intensity of all gravitational waves generated by mergers, as a function of time (Fig. 3). This signal is already several orders of magnitude larger than that observed in today’s Universe by the LIGO/Virgo/Kagra experiments, and is expected to put tough constraints on primordial black holes.
Moreover, the simulations predict that mergers of primordial black holes should already happen in the very young Universe (redshifts 𝑧>100), at a time before any stars have formed. Such a merger could not be explained by astrophysical black holes, and it would be a smoking-gun signal of primordial black holes. Future experiments such as the Einstein Telescope will actually be able to detect such mergers, if primordial black holes in the solar-mass range exist.
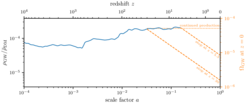