Black hole accretion discs may dance around more slowly than previously thought
Black holes, resulting from the death of massive stars, are some of the most exotic and powerful objects in the Universe. Since even light cannot escape these objects, the quasi-periodic signals coming from the gas falling into the black hole serve as a probe to infer a great deal of information about the black hole and its surrounding environment. The most-commonly observed quasi-periodic signal is thought to originate from the wobbling of hot gas around the black hole, like a spinning top. One problem, though, is that inferred size of this (isolated) corona seemed to be inconsistent with estimations from other observables. With our recent, state-of-the-art computer simulations, involving a more realistic geometry of the accretion flow, we demonstrated for the first time, that the presence of a disc around the corona significantly slows down its precession, relieving much of the tension between this model and observations. These results thus have important implications for studies of black hole properties and how black hole systems form and evolve.
Accretion onto compact objects like black holes, neutron stars or white dwarfs is an important dynamical process that powers many astrophysical sources in the Universe by generating radiative energy from the gravitational potential energy of the accreting matter. Accretion flows are well studied in X-ray binaries (XRBs): close binary systems which usually have a main sequence or a supergiant star as a donor and a neutron star or black hole as an accretor. Matter transferred from the donor star carries certain angular momentum and due to the central gravity force, it gets confined to the orbital plane, forming disk-like structures around the compact object.
Corona around a spinning black hole
Long-term observations have shown that black hole X-ray binaries exhibit strong, aperiodic variability over a broad range of timescales: a few milliseconds to seconds. In addition, these systems also exhibit rapid variability in their X-ray light curves, which are termed quasi-periodic oscillations (QPOs); broadly classified into high-frequency (> 60 Hz) and low-frequency QPOs (< 30 Hz). Understanding the nature of these variabilities and the underlying physical processes helps us probe the nature of the compact object and its surroundings. Apart from the broad picture, very few definite properties are known regarding the origin of the observed variability. This is because the physics and geometry of accreting black holes are highly complex with turbulence, radiation, and 3D effects all coming into play.
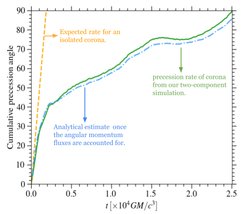
The precession rate computed from our two-component accretion flow simulations (solid, green curve) is significantly lower than the estimated rate for an isolated torus, i.e., not surrounded by an outer, thin disk (dashed, yellow line). When we include the angular momentum flux terms, in addition to the Lense-Thirring torque term, we find remarkable agreement between the simulation results and the analytical estimate (dash-dotted, blue curve).
Using advanced numerical techniques, we performed general relativistic, magneto-hydrodynamic simulations of a truncated disk with an inner, hot flow that is misaligned with the spin axis of the black hole. These simulations are the very first to investigate the effect of the outer, thin disk on the Lense-Thirring precession of the inner, hot accretion flow, and thus add much-needed realism to the usual simulations.
The key finding of our simulations is that the presence of an outer, thin disk decreases the precession rate of the inner torus by nearly 95 per cent. We show that the slowdown in the precession rate is caused by the exchange of the angular momentum between the outer thin and inner thick disks. With this effect, the model now requires a much smaller inner, precessing thick disk to be able to match the typically observed range of type-C QPO frequencies (0.1-10 Hz). Some recent observations have already suggested that the precessing flow needs to be smaller than the estimated size from the Lense-Thirring model for an isolated torus. Thus, our new results help relieve some of the remaining tensions between the model and observations.