Gravitational Wave Messengers from the very early universe
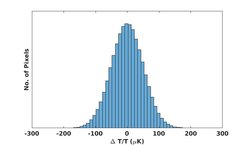
Fig. 1 : Quantum fluctuations produced in the early Universe yield temperature and polarisation fluctuations that obey a Gaussian distribution to high precision.
How did the Universe begin? This profound question has fascinated humans for millennia. Precision cosmology has brought us quite close to answering this question, using observations of the cosmic microwave background (CMB) made by NASA’s WMAP and ESA’s Planck satellites. By making precise measurements of the variation in temperature and polarisation of the CMB over different directions in the sky, cosmologists have zeroed in on a remarkable picture of the origin of cosmic structures such as galaxies, stars, planets, and life: everything around us arose from quantum fluctuations, producing particle-anti particle pairs, in the early Universe. These fluctuations got stretched to very large scales via the accelerated expansion of the Universe, called inflation, in the first fractions of a second. At the end of inflation, the Universe started decelerating and these fluctuations could then seed the present day structures in the Universe.
A key test of the quantum mechanical origin of these fluctuations is their probability distribution, which characterises the probability of the fluctuation having a particular amplitude at a given point in the Universe. For free fields with ground state quantum fluctuations, this distribution is Gaussian (see Fig. 1). However, interactions of the fields with other fields or with themselves in the early Universe make this distribution non-Gaussian. This non-Gaussianity is therefore a crucial probe of interactions of fields during inflation, giving us a window into physics at energy scales much beyond the reach of any particle accelerator on Earth.

Fig. 2 : The 3-point function of gravitational waves produced by vacuum fluctuations of spacetime, which is the correlation between gravitational waves of three different wavelengths, as a function of the ratios of two longer wavelengths to the shortest one. The value in the limit of equal wavelengths (r2 = r3 = 1) corresponds to skewness.
Photons are strongly coupled to electrons until the temperature is sufficiently low to form neutral atoms, which means that the oldest light that we see was emitted when the Universe was already 380 000 years old. Gravitational waves, on the other hand, travel to us almost unimpeded from the beginning of the Universe and are thus a much cleaner probe of inflationary dynamics. While these gravitational waves are difficult to observe directly, they leave their imprint as a unique pattern of polarisation in the CMB.
More precisely, if gravity is quantized, its vacuum fluctuations would produce gravitational waves and hence, a polarisation in the CMB. The amplitude of this polarisation depends on the energy scale of inflation, allowing us to test the reach of our physical theories. However, there is a catch: other sources of energy, such as gauge fields, can produce gravitational waves as well. In this case, the amplitude of the polarisation does not depend simply on the energy scale of inflation, but also on the exact mechanism by which these other fields produce gravitational waves. So, if we observe the imprint of gravitational waves on the CMB polarisation, how can we determine their origin?
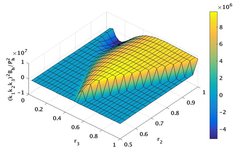
Fig. 3 : Same as Fig. 2 but for gravitational waves generated by interactions of gauge fields. The values are much larger than that for vacuum fluctuations (Fig. 2). The shape is also visibly different from that of vacuum fluctuations.
Scientists at MPA recently established the Gaussianity of gravitational waves as the discerning test of their origin. Using the skewness of the probability distribution (or more precisely, the so-called three-point function) as a measure of non-Gaussianity, they found that while gravitational waves produced by vacuum fluctuations only have a skewness of few times their variance squared (see Fig. 2), those sourced by gauge fields can have a skewness almost a million times their variance squared (see Fig. 3).
This can be easily observed in upcoming CMB missions such as Japan’s LiteBIRD, which greatly improves upon the sensitivities of WMAP and Planck, and in which MPA scientists are also heavily involved. If we find that the observed primordial gravitational waves are indeed highly non-Gaussian, their skewness can be used to measure the energy density fraction of gauge fields during inflation, allowing us to probe the constituents of our Universe when it was less than a trillionth of a trillionth of a trillionth (10-36) of a second old.