Towards a complete model of the interstellar medium
For the first time MPA scientists and European collaborators have simulated the solar neighborhood interstellar medium (ISM), including physical processes following all major thermal and non-thermal components - ionized, neutral and molecular gas, dust, interstellar radiation, magnetic fields, and cosmic rays in the presence of star formation. As the different processes strongly influence each other, the simulations highlight the importance of including them all, in particular radiation and cosmic rays, for a realistic model of the star-forming ISM. Within the Gauss Center for Supercomputing (GCS) project SuperSILCC the team will use SuperMUC-NG, one of the world’s fastest supercomputers, to reveal the physical origins of the ISM structure also in extreme environments at high redshift.
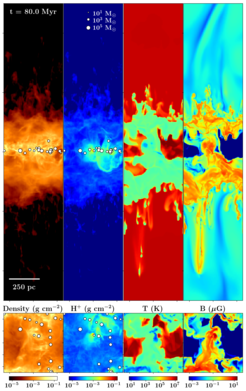
The interstellar medium (ISM) is traditionally defined as everything in-between the stars in galaxies. In fact this accounts for most of the observable baryonic matter in galactic disks like the Milky Way, where star formation takes place and galaxies grow in size and mass. The ISM transitions smoothly to the circum galactic medium (CGM) in the more spherical galactic halos, which can contain significant fractions of the total baryonic mass gravitationally bound to galaxies but shows no evidence for star formation. The CGM is supplied with material outflowing from the ISM, similarly the ISM is replenished by material falling in and cooling from the CGM. Part of the ISM is in the form of ionized, neutral and molecular gas as well as dust. Molecular gas is typically found in structured and compact molecular clouds, where all new stars in galaxies are born. Most of the volume in the ISM, however, is occupied by neutral and ionized gas. Interstellar radiation from stars or gas cooling processes is also part of the ISM. In addition, magnetic fields and cosmic rays – typically protons at relativistic speeds – are energetically equally important non-thermal components.
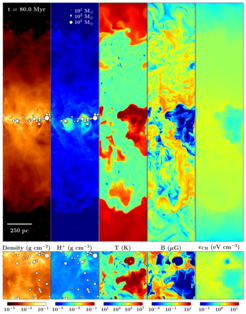
There is a plethora of physical processes regulating the structure and composition of the ISM. Molecular clouds form by cooling and gravitational collapse of the magnetized gas in dust shielded regions. O and B star clusters ionize and heat their surroundings by UV radiation and stellar winds partly dispersing their parental clouds. At the end of their lifetime supernovae drive strong shocks into the turbulent ISM by generating hot ionized gas in expanding super-bubbles, which can escape the ISM in an outflow. Cosmic rays generated in these shocks interact with the magnetic field and generate an additional pressure component driving gas out of the ISM.
As part of the SILCC (SImulating the Life Cycle of molecular Clouds) project the team had shown that the interplay of the above processes is so complex that their inclusion or omission in model calculations can qualitatively change the properties of the ISM and lead to scientifically false outcomes. For example not accounting for the hot gas generated in supernova explosions results in an unrealistic ISM phase structure with just two (warm and cold gas) phases instead of the observed three phases (warm, cold and hot gas); omitting stellar radiation and stellar winds results in too much gas cooling and star formation and too little ionized gas; the presence of magnetic fields delays the collapse into dense structures; and cosmic rays diffusing along magnetic fields can suddenly drive smooth stellar winds transporting material out of the stellar disk into the galactic halo. It has become clear that all major processes have to be included in highly complex computer simulations to develop a comprehensive model of the star-forming ISM. SuperSILCC, the successor of the SILCC project, aims at this new frontier in the numerical modeling of a realistic, turbulent, multi-phase ISM.
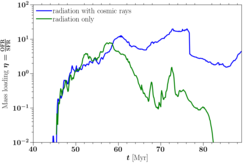
We have now performed the first self-consistent ISM scale simulations of a small patch of a stratified galactic disk accounting for the evolution of all major thermal and non-thermal components following non-equilibrium chemistry, cooling and heating of the dusty magnetized ISM, clustered star formation, ionizing radiation and winds from massive stars, their supernova explosions and the generation and propagation of cosmic rays. The simulations indicate that radiation wind and supernova feedback from massive stars create a turbulent magnetized multi-phase ISM with realistic star formation rates for solar neighborhood conditions as well as the correct (?) structure and abundance of ionized, neutral and molecular gas. (Fig. 1). However, the inclusion of cosmic rays results in the additional generation of a smooth and warm outflow resulting in better agreement with observations of the gas scale heights in the Galactic disk (Fig. 2). The difference in outflow properties can be quantified by the mass-loading factor, i.e. the ratio of gas leaving the ISM in an outflow to gas being converted into new stars in the ISM. It is much higher in the presence of cosmic rays due to their additional pressure gradient (Fig. 3). As of now, this is the most realistic and also physically most complex simulation of a multi-phase turbulent ISM with solar neighborhood conditions.
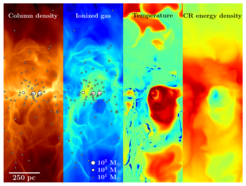
To study these effects in more detail the team has been awarded Tier-0 supercomputing time through the Gauss Center for Supercomputing (GCS) project SuperSILCC on SuperMUC-NG, one of the world’s fastest supercomputers (Top500) at the Leibnitz Supercomputing Center (LRZ). For this project, we will not only investigate the physical origin of the ISM structure on larger scales and in extreme starburst environments at high redshift. We will also simulate the impact of the enigmatic runaway O stars which are observed to leave their birth sites at velocities of several tens of kilometers per second and travel far away from the galactic mid-plane before they explode as supernovae and enrich the ISM with metals (Fig. 4). This process is so far neglected in most ISM simulations and might have a significant impact on the structure of the ISM and the driving of outflows.
Tim-Eric Rathjen & Thorsten Naab for the SuperSILCC team
The GCS SuperSILCC project PIs are:
Philipp Girichidis - AIP, Potsdam
Tim-Eric Rathjen, Thorsten Naab - MPA,
Stefanie Walch, Frantisek Dinnbier, Daniel Seifried - I. Physics Institute, University of Cologne,
Richard Wünsch - Astronomical Institute, Academy of Sciences, Prague
This work is supported by: