Gravitational wave sources in quadruple-star systems
Massive stars often reside in multiple star systems, therefore it is expected that mergers of their final stages, neutron stars or black holes, contribute significantly to gravitational wave sources. A team at MPA has now studied the evolution of millions of quadruple star systems and estimated that a significant fraction of the black hole – black hole mergers detected by LIGO come from such multiple star systems rather than simple binaries.
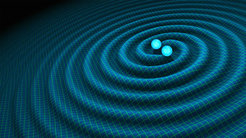
In the past decade, research in gravitational wave astronomy has surged rapidly. Almost a century after Albert Einstein predicted the existence of gravitational waves in 1916, the LIGO observatories in the USA first directly detected such sources in 2015. Since then, the number of known gravitational wave sources has increased, reaching almost a hundred events as of 2022. But what are gravitational waves?
General relativity postulates that matter in the universe curves space-time, and the curvature depends on how dense the matter is. When masses accelerate, this results in ripples or ‘gravitational waves’ in the curvature of space-time, which propagate at the speed of light. These ripples can be detected by the LIGO and Virgo interferometers during very high-energy events, such as the merger of compact objects like neutron stars and black holes.
Neutron stars and black holes are the final stages in the evolution of massive stars, whose masses exceed approximately eight solar masses. For a merger of two such objects to occur, there need to be at least two stars that are gravitationally bound to each other. These two stars need to evolve from the main sequence, survive all the stages of stellar evolution (i.e., do not merge prematurely) and end up as compact objects that merge within the age of the Universe (approximately 14 billion years). The chances of this chain of events occurring are low, which in turn affects the detection rates of gravitational wave events.
Hence, it is crucial to study the evolution of binary stars to be able to understand the progenitors of gravitational waves. Moreover, even higher order multiples, such as triple- and quadruple-star systems, contribute significantly to compact object mergers. This is because massive stars, which eventually become compact objects, mostly reside in multiple star systems. Furthermore, the long-term or ‘secular’ evolution of the dynamics of triples and quadruples can be complex and chaotic, with changes in orbital eccentricities that can lead to mergers.

In this work, we studied the two types of quadruple-star systems: the 2+2 and the 3+1 quadruples (see Fig. 2). Both types are long-term stable and have been observed. Such multiple-star systems are hierarchical in the sense that one can approximate them as nested binaries. A 2+2 quadruple consists of two ‘inner’ binaries which orbit each other, and a 3+1 quadruple consists of one inner binary, orbited by a companion, which in turn is orbited by another companion farther out.
Due to long-term gravitational effects (i.e., secular evolution), these nested orbits are not static but dynamic, meaning that orbital eccentricities can change over time. In our study, we used the code MSE (Multiple Stellar Evolution) developed in our group to evolve 1.6 million quadruple-star systems for 10 billion years. The MSE code self-consistently incorporates prescriptions for single and binary stellar evolution and gravitational dynamics. Binary evolution can involve the transfer of mass between two stars, which might form a common envelope. This event is critical for compact object mergers because friction with the common envelope plays a major role in bringing the two cores (and subsequently neutron stars and black holes) close enough for them to merge within the age of the universe.
We found that most of the mergers are solely due to common envelope evolution, between 70 and 85 % depending on the model assumptions. Long-term gravitational dynamics through secular evolution also plays a role in compact object mergers, as do supernova natal kicks. This is because these kicks almost always result in the unbinding of a binary system and thus prevent a merger from occurring. Supernova kicks also diminish the effect of eccentricity enhancements from secular evolution. Thus, LIGO would probably not be able to observe significant eccentricities during a gravitational wave detection from an initially quadruple-star system. Because of the destructive nature of the kicks, we did not find any system which has more than two bound compact objects during a merger.
Regarding the number of compact object mergers, 2+2 quadruples are much more likely hosts for gravitational wave progenitors than 3+1 quadruples. The reason is twofold. Firstly, 3+1 quadruples have a higher chance of becoming unbound due to dynamical instability. Secondly, 2+2 quadruples have two relatively close inner binaries, either of which can host a potential compact object merger, whereas a 3+1 quadruple only has one inner binary.
The merger rates in the case of two black holes represent a significant fraction of the rates predicted by LIGO, whereas the other merger rates (black hole – neutron star and neutron star – neutron star) fall short of LIGO estimates. This dearth of neutron star mergers is seen in other similar studies as well. It is difficult to replicate LIGO neutron star merger rates due to the high number of (destabilizing) supernova kicks that neutron stars receive. Moreover, the LIGO rates for neutron star – black hole and double neutron star mergers are not well constrained simply because only two each of such events have been detected so far.
The compact object merger rates we found are also comparable to those in binary- and triple-star systems. This confirms our initial expectation that quadruples cannot be ignored in the study of gravitational wave progenitors.